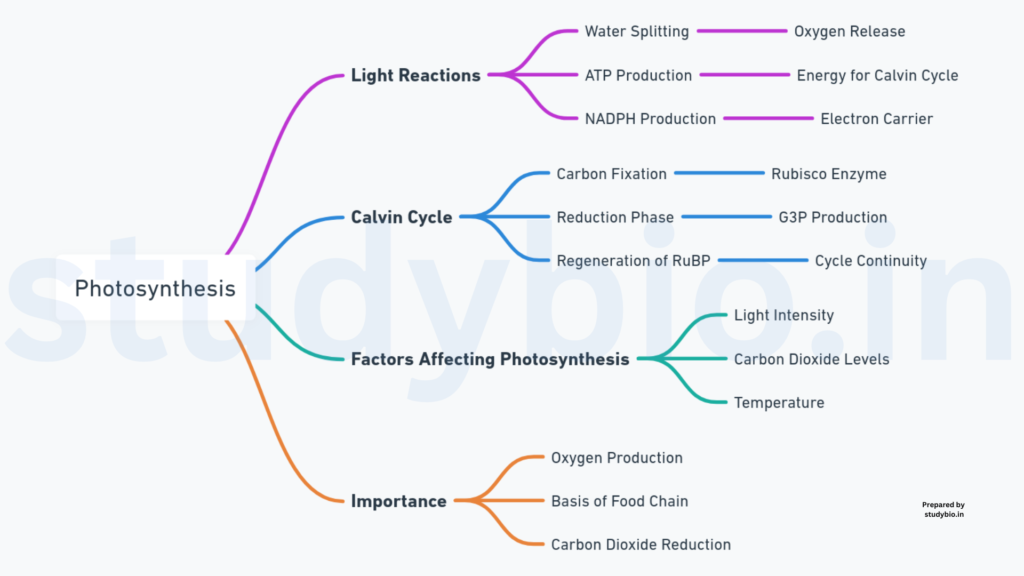
WHAT WE KNOW ABOUT PHOTOSYNTHESIS
Requirements for Photosynthesis:
- Chlorophyll
- Green pigment in the leaf.
- Light
- Essential for photosynthesis.
- CO2 (Carbon Dioxide)
- Necessary for the process.
Experiments and Observations:
- Starch Formation Experiment:
- Procedure:
- Variegated leaf or partially covered leaf exposed to light.
- Testing for starch presence.
- Observation:
- Photosynthesis occurs only in the green parts of the leaves.
- The starch formation was evident in the presence of light.
- Procedure:
- Leaf in Test Tube Experiment:
- Procedure:
- Part of a leaf enclosed in a test tube with KOH-soaked cotton (absorbing CO2).
- The other half is exposed to air.
- Testing for starch presence.
- Observation:
- The exposed part of the leaf tested positive for starch.
- The portion in the tube tested negative.
- CO2 requirement for photosynthesis was demonstrated.
- Procedure:
EARLY EXPERIMENTS IN UNDERSTANDING PHOTOSYNTHESIS
Joseph Priestley’s Experiments (1770):
- Objective:
- Investigate the role of air in the growth of green plants.
- Observations:
- Candle extinguished in a closed space.
- Mouse suffocated in a closed space.
- Mint plant in a bell jar allowed the mouse to stay alive and the candle to burn.
- Hypothesis:
- Plants restore to the air what breathing animals and burning candles remove.
Jan Ingenhousz’s Experiments (Late 18th Century):
- Sunlight and Plant Process:
- Placed setup in sunlight and darkness.
- Sunlight is essential for the plant process purifying air fouled by burning candles or breathing animals.
- Formation of oxygen bubbles around green parts in sunlight.
- Green parts release oxygen.
Julius von Sachs (1854):
- Glucose Production and Chloroplasts:
- Provided evidence for glucose production during plant growth.
- Identified chlorophyll in special bodies (chloroplasts) within plant cells.
- Green parts of plants produce glucose stored as starch.
T.W Engelmann’s Experiments (1843 – 1909):
- Action Spectrum of Photosynthesis:
- Used prism to split light into spectral components.
- Illuminated green alga, Cladophora, in a suspension of aerobic bacteria.
- Bacteria accumulated mainly in the region of blue and red light.
- The first action spectrum of photosynthesis is described, resembling chlorophyll absorption spectra.
Cornelius van Niel (1897-1985):
- Light-Dependent Reaction and Hydrogen Source:
- Studied purple and green bacteria.
- Demonstrated photosynthesis as a light-dependent reaction.
- Hydrogen from an oxidizable compound reduces CO2 to carbohydrates.
- In green plants, H2O is the hydrogen donor, producing O2.
- O2 in green plants comes from H2O, not from CO2.
- The multi-step process is known as photosynthesis, producing glucose ([C6H12O6]) and releasing O2 from water.
LOCATION OF PHOTOSYNTHESIS
Primary Location:
- Green Leaves:
- Main site of photosynthesis.
- Mesophyll cells contain a large number of chloroplasts.
Other Green Parts:
- Other plant parts with chloroplasts:
- Stems
- Petioles
- Green fruits
Chloroplast Orientation:
- Mesophyll Cells:
- Chloroplasts align along mesophyll cell walls.
- Optimal light absorption.
- Flat surfaces parallel to walls when incident light is optimal.
- Perpendicular when incident light is suboptimal.
Chloroplast Structure:
- Components:
- Grana:
- Membranous system.
- Traps light energy.
- Synthesizes ATP and NADPH.
- Stroma Lamellae:
- Membrane structure.
- Stroma:
- Enzymatic reactions.
- Synthesizes sugar, forming starch.
- Grana:
Division of Labour:
- Chloroplast Functions:
- Membrane System:
- Light reactions (photochemical reactions).
- Stroma:
- Dark reactions (carbon reactions).
- Dependent on ATP and NADPH from light reactions.
- Membrane System:
Note: Photosynthesis primarily occurs in green leaves but extends to other green plant parts. Chloroplasts in mesophyll cells play a crucial role, aligning for optimal light absorption. The chloroplast’s structure, with grana and stroma, reflects a division of labor where light reactions occur in the membrane system, while dark reactions, dependent on light products, occur in the stroma. The terms “dark reactions” don’t imply they occur in darkness; they are light-dependent processes.
PLANT PIGMENTS IN PHOTOSYNTHESIS
Types of Pigments:
- Chlorophyll a:
- Bright or blue-green.
- Primary photosynthetic pigment.
- Absorbs light most effectively in the red and violet-blue parts of the spectrum.
- Chlorophyll b:
- Yellow-green.
- Assists chlorophyll a in capturing light energy.
- Broadens the range of light absorption.
- Xanthophylls:
- Yellow.
- Absorb light in the blue region.
- Assist in light absorption.
- Carotenoids:
- Yellow to yellow-orange.
- Broaden the spectrum of light absorption.
- Protect chlorophyll from excessive light.
Leaf Pigment Separation:
- Paper Chromatography:
- Reveals multiple pigments in leaves.
- Colors include bright or blue-green (chlorophyll a), yellow-green (chlorophyll b), yellow (xanthophylls), and yellow to yellow-orange (carotenoids).
Role of Pigments:
- Chlorophyll a:
- Main player in photosynthesis.
- Absorbs light in the red and violet-blue spectrum.
- Chlorophyll b:
- Assists chlorophyll a.
- Expands the range of light absorption.
- Xanthophylls:
- Assist in light absorption.
- Contribute to yellow color.
- Carotenoids:
- Broaden light absorption.
- Protect chlorophyll from excessive light.
Abundance:
- Most Abundant Plant Pigment:
- Chlorophyll a:
- Most abundant pigment globally.
- Chlorophyll a:
Light Absorption:
- Chlorophyll and Absorption:
- Highest absorption in the red and violet-blue parts.
- Visible spectrum: VIBGYOR.
LIGHT REACTIONS IN PHOTOSYNTHESIS
Definition:
- Light Reactions:
- Also known as the ‘Photochemical’ phase.
- Includes light absorption, water splitting, oxygen release, and the formation of high-energy intermediates (ATP and NADPH).
Process Overview:
- Key Steps:
- Light Absorption:
- Pigments absorb light.
- Initiated by two discrete photochemical light-harvesting complexes (LHC) within Photosystem I (PS I) and Photosystem II (PS II).
- Water Splitting:
- Water molecules are split.
- Oxygen is released as a byproduct.
- High-Energy Intermediates:
- Formation of ATP and NADPH.
- Energy carriers for subsequent steps in photosynthesis.
- Light Absorption:
Protein Complexes:
- Photosystems:
- PS I (Photosystem I)
- Absorption peak at 700 nm.
- Designated as P700.
- PS II (Photosystem II)
- Absorption maxima at 680 nm.
- Designated as P680.
- PS I (Photosystem I)
Pigment Organization:
- Light Harvesting Complexes (LHC):
- Hundreds of pigment molecules are bound to proteins.
- Organized within antennae in both PS I and PS II.
Efficiency Enhancement:
- Antennae Function:
- Enhance efficiency by absorbing different wavelengths of light.
- Improve the overall effectiveness of photosynthesis.
Note: Light reactions involve key processes such as light absorption, water splitting, oxygen release, and the generation of high-energy intermediates (ATP and NADPH). Photosystems (PS I and PS II) and light-harvesting complexes (LHC) play vital roles in these reactions, with pigments organized to optimize light absorption. The reaction centers in PS I (P700) and PS II (P680) have absorption peaks at 700 nm and 680 nm, respectively.
ELECTRON TRANSPORT IN PHOTOSYNTHESIS
Photosystem II (PS II):
- Absorption:
- Reaction center chlorophyll-a absorbs 680 nm wavelength of red light.
- Electrons become excited, moving to a higher orbit.
- Electron Movement:
- Excited electrons are transferred to an electron acceptor.
- Passed through an electron transport system consisting of cytochromes.
- Movement is downhill in terms of redox potential.
Photosystem I (PS I):
- Absorption:
- Reaction center chlorophyll-a absorbs 700 nm wavelength of red light.
- Electrons become excited and transferred to another acceptor.
- Electron Movement:
- Electrons move downhill to NADP+.
- NADP+ is reduced to NADPH + H+.
Z Scheme:
- Electron Transfer Path:
- Sequential transfer from PS II to PS I.
- Excitation, transfer, and reduction of NADP+ characterize the Z scheme.
- Shaped by the arrangement of carriers on a redox potential scale.
Water Splitting:
- Association with PS II:
- PS II supplies electrons continuously by replacing those moved to PS I.
- Electrons for replacement are obtained from the splitting of water.
- Water splits into 2H+, [O], and electrons.
- Oxygen is produced as a byproduct.
- Water Splitting Complex:
- Associated with PS II.
- Located on the inner side of the thylakoid membrane.
Note: Photosystem II absorbs 680 nm light, initiating the movement of electrons through an electron transport system. Photosystem I absorbs 700 nm light, and electrons move downhill to reduce NADP+ to NADPH + H+. The Z scheme describes this sequential electron transfer. Water splitting in PS II provides electrons to replace those moved to PS I, generating oxygen as a byproduct. The water-splitting complex is associated with PS II on the inner side of the thylakoid membrane.
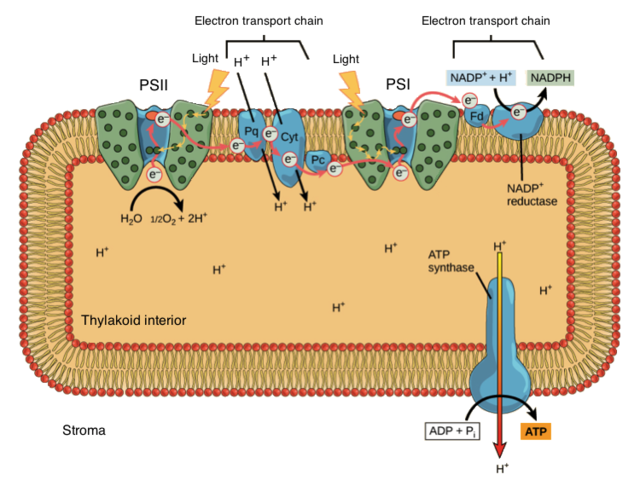
CYCLIC AND NON-CYCLIC PHOTO-PHOSPHORYLATION
Phosphorylation in Cellular Energy Synthesis:
- ATP Synthesis:
- Living organisms extract energy and store it as bond energy.
- Special substances like ATP carry energy in their chemical bonds.
- Phosphorylation Process:
- Phosphorylation is the synthesis of ATP from ADP and inorganic phosphate.
- Photophosphorylation occurs in the presence of light, specifically in chloroplasts.
Non-cyclic Photo-phosphorylation:
- Photosystem Series:
- PS II and PS I work sequentially in non-cyclic photo-phosphorylation.
- The electron transport chain connects the two photosystems (Z scheme).
- Both ATP and NADPH + H+ are synthesized.
Cyclic Photo-phosphorylation:
- Functional PS I Only:
- In cyclic photo-phosphorylation, only PS I is functional.
- Electron circulates within PS I without passing to NADP+.
- Electrons cycled back to PS I through the electron transport chain.
- Location:
- Possibly occurs in the stroma lamellae.
- Stroma lamellae lack PS II and NADP reductase enzymes.
- Result:
- Cyclic flow results in ATP synthesis but not NADPH + H+.
- Occurs when only light beyond 680 nm is available for excitation.
Note: Photophosphorylation is the synthesis of ATP from ADP and inorganic phosphate in the presence of light. Non-cyclic photo-phosphorylation involves both PS II and PS I, connected by an electron transport chain. In cyclic photo-phosphorylation, only PS I is functional, and the electron is cycled back to PS I, resulting in ATP synthesis without NADPH + H+. Cyclic photo-phosphorylation can occur when light beyond 680 nm is available for excitation.
CHEMIOSMOTIC HYPOTHESIS
- The chemiosmotic hypothesis explains ATP synthesis in chloroplasts during photosynthesis.
- Proton gradient across thylakoid membranes plays a crucial role.
Proton Accumulation and Movement:
- Water Splitting:
- Protons produced by the splitting of water accumulate in the thylakoid lumen.
- Electron Transport:
- Electrons’ movement through photosystems causes proton transport across the membrane.
- Primary electron acceptor transfers electrons to an H carrier, releasing protons into the thylakoid lumen.
- NADP Reductase:
- Protons, along with electrons from PS I, are used for NADP+ reduction on the stroma side.
- Protons are removed from the stroma, leading to a decrease in stromal protons and an accumulation in the thylakoid lumen.
Proton Gradient Significance:
- Proton gradient across thylakoid membrane and decreased pH in the lumen are crucial.
- Breakdown of this gradient leads to ATP synthesis.
ATP Synthase Structure and Function:
- ATP synthase consists of two parts:
- CF0: Embedded in the thylakoid membrane, forms a transmembrane channel for facilitated diffusion of protons.
- CF1: Protrudes on the thylakoid membrane’s outer surface facing the stroma.
Energy Conversion:
- Breakdown of the proton gradient causes a conformational change in CF1 of ATP synthase.
- This change activates ATP synthase to synthesize multiple ATP molecules.
Chemiosmosis Process:
- Requirements:
- Membrane
- Proton pump
- Proton gradient
- ATP synthase
- Mechanism:
- Energy pumps protons across the membrane, creating a high concentration in the thylakoid lumen.
- ATP synthase allows proton diffusion back across the membrane.
- Energy released activates ATP synthase, catalyzing ATP formation.
Utilization of ATP and NADPH:
- ATP and NADPH produced during photosynthesis are immediately used in stromal biosynthetic reactions.
- These reactions involve CO2 fixation and sugar synthesis.
ATP and NADPH Utilization in Biosynthetic Phase
In the biosynthetic phase of photosynthesis, ATP and NADPH, products of the light reaction, are utilized to drive processes leading to the synthesis of sugars.
Dependency on Light Reaction Products
- Products of Light Reaction: ATP, NADPH, and O2.
- Oxygen Diffusion: O2 diffuses out of the chloroplast.
- Utilization of ATP and NADPH: Drive processes for synthesizing food (sugars).
Biosynthetic Phase Characteristics
- Independence from Light: The biosynthetic phase does not directly depend on light but relies on light-produced substances.
- Verification of Dependency: When light becomes unavailable, the biosynthetic process continues for a period and resumes upon reintroducing light.
Dark Reaction Misnomer Discussion
- Consideration: Discuss whether labeling the biosynthetic phase as the “dark reaction” is a misnomer.
- Observation: The process occurs in the absence of light but depends on light-produced substances.
Calvin Cycle: CO2 Fixation
- Initiation of CO2 Fixation: CO2 is combined with H2O to produce (CH2O)n or sugars.
- Scientific Contribution: Melvin Calvin’s work, utilizing radioactive 14C in algal photosynthesis studies after World War II.
- First Product: 3-phosphoglyceric acid (PGA).
- Carbon Atoms: PGA is a 3-carbon organic acid.
C3 and C4 Pathways
- CO2 Fixation Types:
- C3 Pathway: First product is PGA (3-carbon acid).
- C4 Pathway: The first product is oxaloacetic acid (OAA) with 4 carbon atoms.
- Scientific Discovery: Experimentation across a variety of plants identified these two main types of CO2 assimilation during photosynthesis.
Primary Acceptor of CO2 in Calvin Cycle
In the Calvin Cycle, the primary acceptor of CO2 is a 5-carbon ketose sugar known as ribulose bisphosphate (RuBP).
Scientific Inquiry
- Question Posed: How many carbon atoms would a molecule fixing CO2 and yielding a 3-carbon product (PGA) have?
- Surprising Discovery: The acceptor molecule was identified as a 5-carbon ketose sugar—RuBP.
- Scientific Exploration: Extensive experiments and prolonged research were conducted to unveil this unexpected result.
Assumption and Exploration
- Initial Assumption: Scientists initially believed the primary acceptor would be a 2-carbon compound, anticipating a 3-carbon product.
- Years of Investigation: The quest to identify a 2-carbon compound continued for years before the 5-carbon RuBP was discovered.
The Calvin Cycle
The Calvin Cycle, elucidated by Calvin and colleagues, operates in a cyclic manner, ensuring the regeneration of ribulose bisphosphate (RuBP).
Pathway Overview
- Universal Occurrence: The Calvin pathway is present in all photosynthetic plants, irrespective of their C3 or C4 pathways.
- Three Stages: The Calvin cycle can be delineated into three stages: Carboxylation, Reduction, and Regeneration.
Stages of the Calvin Cycle
- Carboxylation:
- Definition: Fixation of CO2 into a stable organic intermediate.
- Key Enzyme: RuBP carboxylase catalyzes this crucial step, leading to the formation of two molecules of 3-phosphoglyceric acid (3-PGA).
- Note: RuBP carboxylase is alternatively known as RuBP carboxylase-oxygenase or RuBisCO due to its dual activity.
- Reduction:
- Series of reactions resulting in glucose formation.
- Requirements: Two molecules of ATP for phosphorylation and two of NADPH for reduction per CO2 fixed.
- Glucose Synthesis: Six CO2 molecules and 6 turns of the cycle are necessary for one glucose molecule formation.
- Regeneration:
- Vital for continuous cycle operation.
- Regeneration Steps: One ATP is required for phosphorylation to regenerate the CO2 acceptor molecule RuBP.
- Balance: Each CO2 entering the cycle necessitates 3 ATP and 2 NADPH.
Glucose Synthesis
- Requirements: To produce one molecule of glucose through the Calvin pathway, six turns of the cycle are essential.
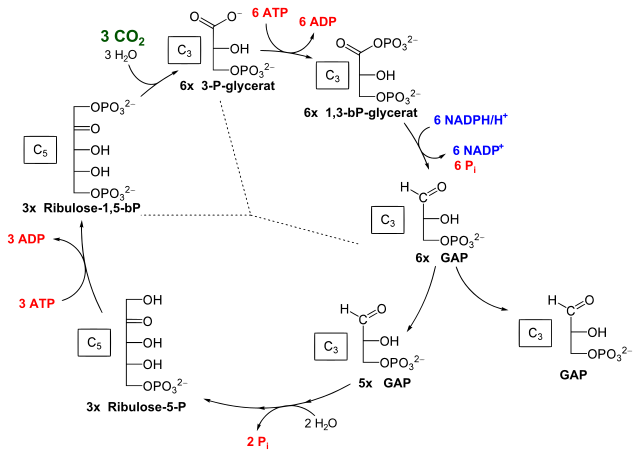
The C4 Pathway
Plants adapted to dry tropical regions employ the C4 pathway, featuring C4 oxaloacetic acid as the initial CO2 fixation product. Despite this, these plants predominantly utilize the C3 pathway or the Calvin cycle for biosynthesis.
Characteristics of C4 Plants
- Leaf Anatomy:
- Differences: Vertical leaf sections of C3 and C4 plants showcase distinctions.
- Mesophyll Cells: C4 plants exhibit particularly large bundle sheath cells around vascular bundles.
- Kranz Anatomy: Leaves with this arrangement are characterized by a wreath-like structure.
- Bundle Sheath Cells: Have numerous chloroplasts, thick impermeable walls, and lack intercellular spaces.
- Hatch and Slack Pathway:
- CO2 Acceptor: Phosphoenolpyruvate (PEP), a 3-carbon molecule in mesophyll cells.
- Enzyme: PEP carboxylase is responsible for CO2 fixation in mesophyll cells.
- Mesophyll Cells: Lack RuBisCO enzyme; C4 acid oxaloacetic acid (OAA) forms in these cells.
- Transport: OAA converts to 4-carbon compounds (malic or aspartic acid) in mesophyll cells and is transported to bundle sheath cells.
- Bundle Sheath Cells: C4 acids break down, releasing CO2 and a 3-carbon molecule.
- Cycle Completion: The 3-carbon molecule returns to mesophyll, converting back to PEP, completing the cycle.
- Calvin Pathway: CO2 released in bundle sheath cells enters the common Calvin pathway.
- Hatch and Slack Pathway Significance:
- RuBisCO Presence: Bundle sheath cells rich in RuBisCO, lacking PEPcase.
- Sugar Formation: Calvin pathway, common to C3 and C4 plants, occurs in bundle sheath cells of C4 plants, not in mesophyll cells.
Photorespiration
Understanding photorespiration sheds light on a significant difference between C3 and C4 plants. Photorespiration is intricately linked to the initial step of the Calvin pathway, where RuBP combines with CO2 to form two molecules of 3PGA catalyzed by RuBisCO.
RuBisCO Characteristics
- Binding Affinity:
- Dual Affinity: RuBisCO’s active site can bind to both CO2 and O2.
- Competitive Binding: Affinity depends on the relative concentrations of O2 and CO2.
- C3 Plants – Photorespiration:
- Occurrence: Some O2 binds to RuBisCO in C3 plants, decreasing CO2 fixation.
- Result: RuBP, instead of forming 3PGA, binds with O2, leading to phosphoglycerate and phosphoglycolate (2 Carbon) in photorespiration.
- Outcome: No sugar or ATP synthesis; CO2 is released with ATP consumption.
- Biological Function of Photorespiration:
- Unknown: The purpose of photorespiration remains unclear in terms of biological function.
- C4 Plants – Photorespiration Absence:
- Mechanism: C4 plants prevent photorespiration by increasing CO2 concentration at the enzyme site.
- Process: C4 acid breakdown in bundle sheath cells releases CO2, elevating intracellular CO2 concentration.
- Result: RuBisCO functions as a carboxylase, minimizing oxygenase activity, and avoiding photorespiration.
Factors Affecting Photosynthesis
Understanding the factors influencing photosynthesis is crucial for determining plant yield, especially in crop plants. These factors can be categorized into internal (plant-related) and external factors.
Internal Factors (Plant-related)
- Leaf Characteristics:
- Number, Size, and Age: Influence the overall photosynthetic capacity.
- Orientation: Affects the exposure of leaves to sunlight.
- Cell and Chloroplast Factors:
- Mesophyll Cells: Their number and efficiency impact photosynthesis.
- Chloroplasts: Their abundance and health are critical for efficient light absorption.
- Internal CO2 Concentration: The availability of carbon dioxide within the plant.
Blackman’s Law of Limiting Factors
- Principle: When multiple factors influence a chemical process like photosynthesis, the rate is determined by the factor closest to its minimal value.
- Example: Even with optimal light and CO2 conditions, if the temperature is very low, it can limit photosynthesis.
Light as a Factor in Photosynthesis
Understanding the impact of light on photosynthesis involves considering light quality, intensity, and duration as distinct factors.
Light Quality
- Definition: Refers to the specific wavelengths of light.
- Importance: Different pigments absorb light at different wavelengths, influencing the efficiency of photosynthesis.
Light Intensity
- Linear Relationship: A linear correlation exists between incident light and CO2 fixation rates at low light intensities.
- Saturation: At higher light intensities, the photosynthetic rate reaches saturation, and further increases are limited due to other factors.
- Threshold: Light saturation occurs at 10% of full sunlight, making light rarely a limiting factor in nature.
Duration of Light Exposure
- Significance: The total duration of light exposure affects the overall photosynthetic output.
- Role: Duration complements light intensity and quality, impacting the rate of photosynthesis.
Limiting Factors
- Beyond Saturation: Excessive light can lead to chlorophyll breakdown, causing a decline in photosynthesis.
- Rare Limitation: In natural conditions, light is seldom a limiting factor except in shaded areas or dense forests.
Carbon Dioxide Concentration in Photosynthesis
Importance of Carbon Dioxide
- Major Limiting Factor: Carbon dioxide (CO2) is the primary limiting factor for photosynthesis.
- Atmospheric Concentration: Atmospheric CO2 concentration is low, ranging between 0.03 and 0.04 percent.
- Optimal Increase: An increase in CO2 concentration up to 0.05 percent enhances CO2 fixation rates, but beyond this, it can become damaging over extended periods.
Response of C3 and C4 Plants
- Differential Responses: C3 and C4 plants exhibit distinct responses to CO2 concentrations.
- Light Dependency: At low light conditions, neither C3 nor C4 plants respond significantly to elevated CO2 levels.
- High Light Intensities: Both C3 and C4 plants demonstrate increased photosynthetic rates under high light intensities.
- Saturation Levels: C4 plants saturate at approximately 360 µL-1 CO2, whereas C3 plants respond positively to increased CO2 concentration, saturating beyond 450 µL-1.
Practical Applications
- Greenhouse Crops: C3 plants, like tomatoes and bell peppers, benefit from increased CO2 concentration.
- Enhanced Yields: Growing these crops in a carbon dioxide-enriched atmosphere results in higher yields due to increased photosynthetic rates.
Temperature in Photosynthesis
Temperature Sensitivity
- Enzymatic Control: Dark reactions in photosynthesis are enzymatic and strongly temperature-controlled.
- Light Reactions: Light reactions are also temperature-sensitive, but their sensitivity is comparatively lower.
Differential Responses in C3 and C4 Plants
- Temperature Influence: C4 plants exhibit a more favorable response to higher temperatures, resulting in increased photosynthetic rates.
- Temperature Optimum: C3 plants, on the other hand, have a lower temperature optimum for photosynthesis.
Adaptation to Habitat
- Habitat Dependence: The temperature optimum for photosynthesis varies among plants based on their adaptation to different habitats.
- Tropical Plants: Plants adapted to tropical climates generally have a higher temperature optimum compared to those adapted to temperate climates.
Water in Photosynthesis
Indirect Impact on Photosynthesis
- Reactant in Light Reaction: Water is a crucial reactant in the light reaction of photosynthesis.
- Indirect Influence: The impact of water on photosynthesis is more indirect, affecting the plant’s overall physiological conditions.
Stomatal Regulation
- Stomatal Closure: Water stress leads to stomatal closure, limiting the entry of CO2 into the leaves.
- Reduced CO2 Availability: Closed stomata reduce the availability of CO2 for the photosynthetic process.
Wilted Leaves and Metabolic Activity
- Leaf Wilting: Water stress causes leaves to wilt, reducing their turgor pressure.
- Surface Area Reduction: Wilting results in a reduction of the leaf surface area, affecting the overall metabolic activity of the leaves.
Abbreviations:
ADP | adenosine diphosphate |
ATP | adenosine triphosphate |
CH2O | carbohydrate |
cytb6f | cytochrome b6f |
DHAP | dihydroxyacetone phosphate |
EET | excitation energy transfer |
FNR | ferredoxin–NADP+ reductase |
GAP | glyceraldehyde 3-phosphate |
LHC | light-harvesting complex |
NADPH | nicotinomide–adenine dinucleotide phosphate |
PEP | phosphoenolpyruvate |
Pi | inorganic phosphate |
PSI | Photosystem I |
PSII | Photosystem II |
RC | reaction centre |
Rubisco | ribulose-1,5-bisphosphate carboxylase/oxygenase |