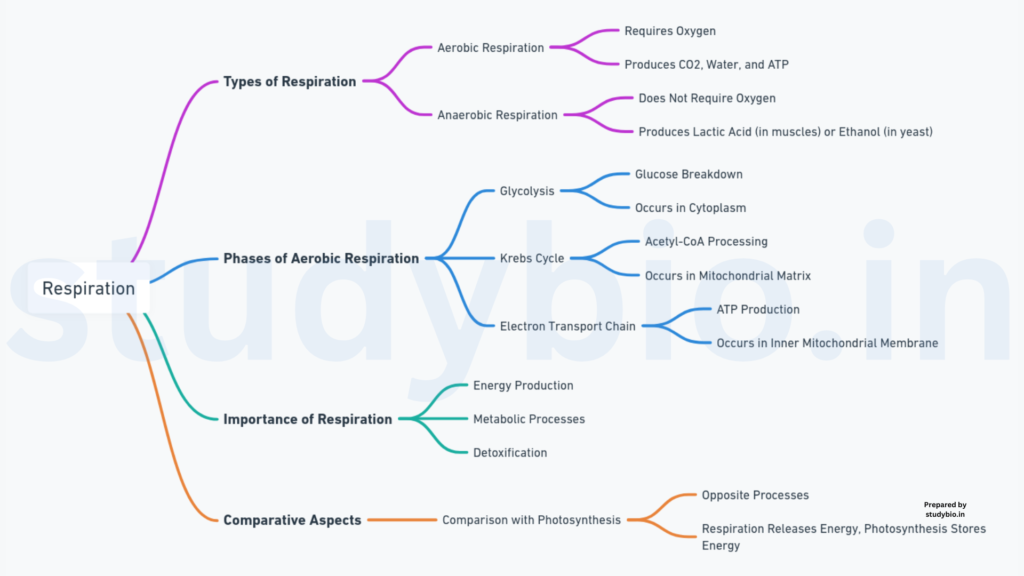
Introduction
- Life Processes and Energy: All living organisms require energy for essential life processes.
- Energy Sources: Energy is obtained through the oxidation of macromolecules known as “food.”
Photosynthesis and Food Production
- Green Plants and Cyanobacteria: Capable of photosynthesis, converting light energy into chemical energy stored in carbohydrates (glucose, sucrose, starch).
- Food Translocation: Not all cells in green plants photosynthesize; food must be translocated to non-green parts.
Heterotrophic Organisms
- Animal and Fungal Nutrition: Animals (herbivores, carnivores) obtain food directly or indirectly from plants. Fungi are saprophytes, relying on dead and decaying matter.
- Ultimate Source: Regardless of the organism’s nutritional strategy, the ultimate source of food for respiration is photosynthesis.
Cellular Respiration Process
- Cellular Locations: Photosynthesis occurs in chloroplasts, while cellular respiration takes place in the cytoplasm and mitochondria (eukaryotes).
- C-C Bond Breakdown: Respiration involves the breakdown of C-C bonds through oxidation, releasing energy.
- Respiratory Substrates: Carbohydrates are commonly oxidized, but proteins, fats, and organic acids may also serve as respiratory substrates in specific conditions.
ATP Synthesis and Utilization
- Series of Reactions: Energy release in respiration occurs in a series of controlled, enzymatic reactions.
- ATP Synthesis: Energy released is trapped as chemical energy in the form of ATP.
- Energy Currency: ATP serves as the energy currency of the cell, utilized in various energy-requiring processes.
- Carbon Skeleton Utilization: Carbon skeletons produced during respiration serve as precursors for the biosynthesis of other molecules in the cell.
- Photosynthesis Adaptations: During photosynthesis, O2 release within cells addresses O2 availability.
Gaseous Exchange Mechanisms
- Cell Proximity to Surface: Living cells in plants are close to the surface, facilitating efficient gas exchange.
- Parenchyma Cells: Loose packing of parenchyma cells in leaves, stems, and roots creates interconnected air spaces.
Energy Production and Utilization
- Complete Glucose Combustion: Glucose combustion produces CO2, H2O, and energy (mostly as heat).
- Energy Utilization Strategy: Cells catabolize glucose in multiple small steps, coupling energy release to ATP synthesis.
- Story of Respiration: Respiration involves oxygen utilization, releasing CO2, water, and energy.
Anaerobic Adaptations
- Oxygen Availability: Some cells live in environments with varying oxygen availability.
- Adaptation to Anaerobic Conditions: Early cells likely existed in oxygen-lacking atmospheres; present-day organisms adapted to anaerobic conditions.
Glycolysis: Anaerobic Glucose Breakdown
- Enzymatic Machinery: Living organisms retain enzymatic machinery for partial glucose oxidation without oxygen.
- Glycolysis: Breakdown of glucose to pyruvic acid in the absence of oxygen.
Glycolysis: Breaking Down Glucose for Energy
Introduction
- Origin of the Term: “Glycolysis” from Greek words glycos (sugar) and lysis (splitting).
- Pioneers: Gustav Embden, Otto Meyerhof, and J. Parnas developed the glycolysis scheme.
- Anaerobic Process: Predominant in anaerobic organisms; occurs in the cytoplasm.
Glycolytic Pathway in Plants
- Glucose Source: Derived from sucrose (photosynthesis end product) or storage carbohydrates.
- Sucrose Conversion: Invertase converts sucrose into glucose and fructose.
- Initial Steps: Glucose and fructose phosphorylation to form glucose-6-phosphate.
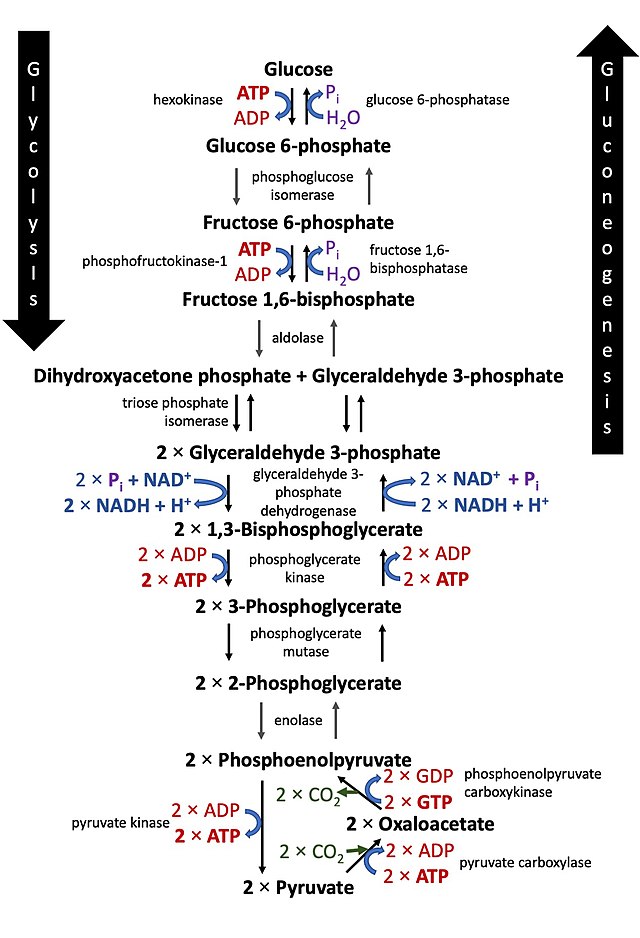
Ten Steps of Glycolysis
- Hexokinase Activity: Glucose to glucose-6-phosphate.
- Isomerization: Glucose-6-phosphate to fructose-6-phosphate.
- Common Metabolism: Subsequent metabolism steps for glucose and fructose are the same.
- ATP Utilization: Twice during glucose conversion and fructose-6-phosphate conversion.
- Fructose 1,6-Bisphosphate Formation: ATP consumption.
- Bisphosphate Split: Fructose 1,6-bisphosphate to dihydroxyacetone phosphate and 3-phosphoglyceraldehyde.
NADH + H+ Formation
- Redox Reaction: 3-phosphoglyceraldehyde to 1, 3-bisphosphoglycerate.
- Hydrogen Transfer: NADH + H+ formation.
ATP Synthesis
- Energy Yielding Steps: Conversion of 1, 3-bisphosphoglycerate to 3-phosphoglyceric acid.
- ATP Formation: During the conversion of PEP to pyruvic acid.
Overall ATP Calculation
- Direct ATP Synthesis: Calculate the total ATP molecules directly synthesized from one glucose molecule.
Pyruvic Acid as a Product
- Metabolic Fate: Determined by cellular needs.
- Possibilities: Lactic acid fermentation, alcoholic fermentation, or aerobic respiration.
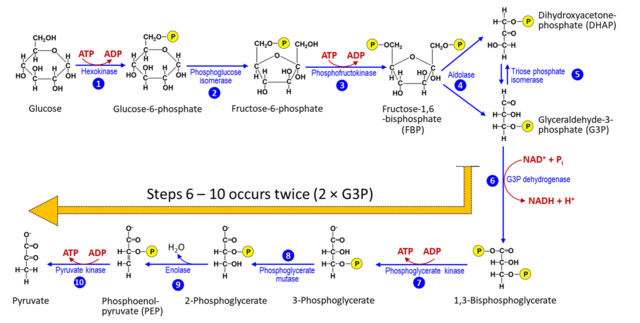
Fermentation: Anaerobic Energy Production
Overview
- Definition: Incomplete oxidation of glucose under anaerobic conditions.
- Byproducts: CO2 and ethanol (yeast), or lactic acid (bacteria and muscles).
- Enzymes: Pyruvic acid decarboxylase and alcohol dehydrogenase (for alcohol fermentation).
Yeast Fermentation
- Pyruvic Acid Conversion: Catalyzed by pyruvic acid decarboxylase.
- Ethanol Formation: Through alcohol dehydrogenase.
- Energy Release: Limited energy release (less than 7% of glucose energy).
- ATP Synthesis: Not highly efficient; some ATP formed.
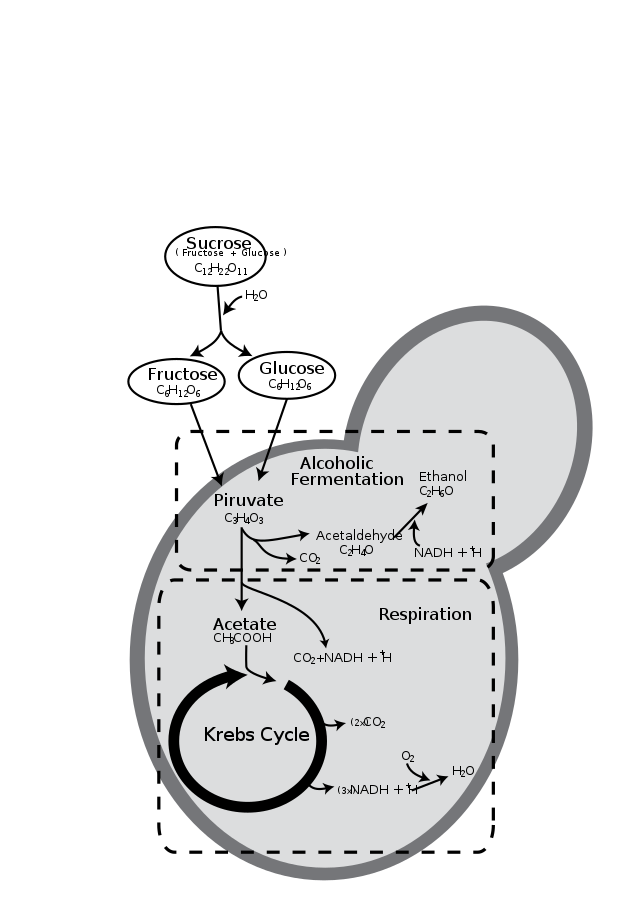
Lactic Acid Fermentation
- Bacterial Process: Some bacteria convert pyruvic acid to lactic acid.
- Animal Cells: Muscles during anaerobic conditions convert pyruvic acid to lactic acid.
- NADH+H+ Reoxidation: NAD+ regeneration via reduction of pyruvic acid.
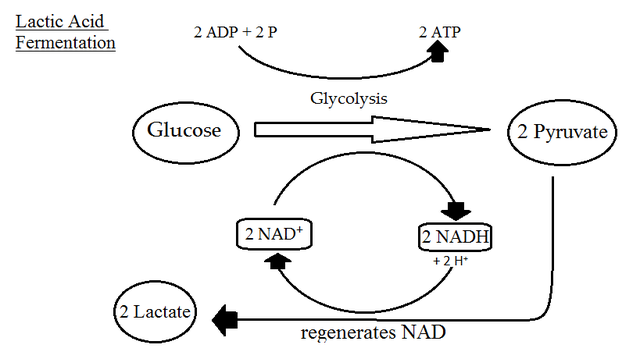
Energy Yield and Hazards
- Limited ATP Production: Not all energy is trapped as high-energy ATP bonds.
- Hazardous Byproducts: Production of acid (lactic acid) or alcohol.
- Yeast Poisoning: Yeasts are affected when alcohol concentration reaches about 13%.
Net ATP Synthesis
- Calculation: Deduct ATP used during glycolysis from ATP synthesized during fermentation.
Maximum Alcohol Concentration
- Yeast Limitation: Yeasts poison themselves at high alcohol concentrations.
- Natural Fermentation: Maximum alcohol content limited by yeast tolerance.
Complete Oxidation in Aerobic Respiration
- Mitochondrial Steps: Complete oxidation occurs within mitochondria.
- Oxygen Dependency: Requires oxygen (O2).
- Products: CO2, water, and substantial energy release for ATP synthesis.
- Prevalence: Common in higher organisms.
Aerobic Respiration: Mitochondrial Processes
Overview
- Location: Mitochondria (matrix and inner membrane).
- Pyruvate Transport: From cytoplasm to mitochondria.
- Crucial Events:
- Oxidation of Pyruvate:
- Removal of all hydrogen atoms.
- Production of three molecules of CO2.
- Occurs in the matrix of mitochondria.
- Electron Transfer to O2:
- Electrons passed to molecular O2.
- Simultaneous ATP synthesis.
- Located on the inner membrane of mitochondria.
- Oxidation of Pyruvate:
Pyruvate Oxidation
- Pyruvate Formation: Glycolytic catabolism in cytosol produces pyruvate.
- Transport to Mitochondria: Pyruvate enters mitochondrial matrix.
- Oxidative Decarboxylation: Catalyzed by pyruvic dehydrogenase.
- Coenzymes: Involves NAD+ and Coenzyme A.
- Reaction:
NADH Production
- NADH Synthesis: Two molecules of NADH from the metabolism of two pyruvic acid molecules (from one glucose during glycolysis).
Tricarboxylic Acid Cycle (TCA Cycle)
Overview
- Start: Condensation of acetyl group with oxaloacetic acid (OAA) and water.
- Enzyme: Catalyzed by citrate synthase.
- Product: Citric acid and release of CoA.
- Isomerization: Citrate is isomerized to isocitrate.
- Decarboxylation Steps:
- Formation of α-ketoglutaric acid.
- Formation of succinyl-CoA.
- Oxidation Steps:
- Succinyl-CoA to succinic acid.
- GTP synthesis in substrate-level phosphorylation.
- GTP converted to GDP, producing ATP.
- Reduction Steps:
- Three points where NAD+ is reduced to NADH + H+.
- One point where FAD+ is reduced to FADH2.
- Continued Cycle: Requires replenishment of oxaloacetic acid and regeneration of NAD+ and FAD+.
Substrate-Level Phosphorylation
- Process: Conversion of succinyl-CoA to succinic acid.
- Result: Synthesis of one molecule of GTP.
- Coupled Reaction: GTP converted to GDP, simultaneously synthesizing ATP from ADP.
NADH and FADH2 Production
- NADH Production: Three points in the cycle.
- FADH2 Production: One point in the cycle.
Cycle Significance
- Purpose: Continued oxidation of acetyl CoA.
- Requirements:
- Replenishment of oxaloacetic acid.
- Regeneration of NAD+ and FAD+ from NADH and FADH2.
- Products So Far: CO2, eight NADH + H+, two FADH2, and two ATP.
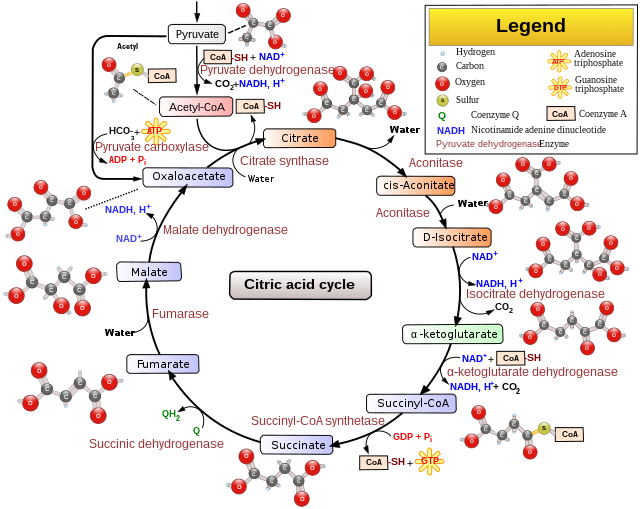
Electron Transport System (ETS) and Oxidative Phosphorylation
Overview
- Objective: Release and utilize the energy stored in NADH+H+ and FADH2.
- Location: Inner mitochondrial membrane.
- Process: Oxidation of NADH and FADH2 through the electron transport system (ETS).
- Result: Formation of H2O, coupled with ATP synthesis.
Electron Transport System (ETS) Components
- NADH Dehydrogenase (Complex I):
- Oxidizes electrons from NADH produced in the mitochondrial matrix.
- Transfers electrons to ubiquinone.
- Ubiquinone:
- Receives electrons from NADH dehydrogenase (complex I) and FADH2 (complex II).
- Transfers electrons to cytochrome c.
- Cytochrome c and Cytochrome bc1 Complex (Complex III):
- Cytochrome c acts as a mobile carrier.
- Receives electrons from reduced ubiquinone.
- Transfers electrons to cytochrome c oxidase complex (complex IV).
- Cytochrome c Oxidase Complex (Complex IV):
- Contains cytochromes a and a3, and two copper centers.
- Accepts electrons from cytochrome c.
- Transfers electrons to oxygen, forming H2O.
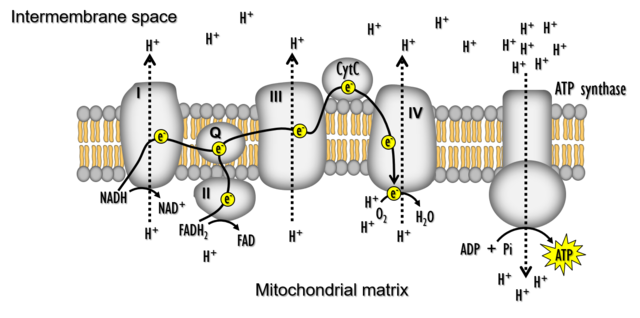
ATP Synthesis
- Coupled with ETS: ATP synthase (complex V) couples with the electron transport chain (ETS).
- Components:
- F1 Headpiece: Peripheral membrane protein complex with ATP synthesis site.
- F0 Integral Membrane Protein: Forms a channel for proton movement.
- Proton Movement: Protons move through F0 from the intermembrane space to the matrix down the electrochemical proton gradient.
- Proton Passage Coupling: Coupled to the catalytic site of the F1 component for ATP production.
- ATP Synthesis: For each ATP produced, 4H+ passes through F0.
Role of Oxygen
- Terminal Stage: Oxygen acts as the final hydrogen acceptor.
- Importance: Drives the entire process by removing hydrogen from the system.
- Role: Oxygen is the final electron acceptor, forming water.
Oxidative Phosphorylation
- Definition: The process is termed oxidative phosphorylation due to the utilization of energy from oxidation-reduction for ATP synthesis.
- Comparison to Photophosphorylation: Unlike photophosphorylation, where light energy is utilized, oxidative phosphorylation uses the energy from oxidation-reduction.
ATP Synthase (Complex V)
- Components:
- F1 Headpiece: Peripheral membrane protein complex.
- F0 Integral Membrane Protein: Forms the proton channel.
- Energy Source: Energy released during the electron transport system.
- Function: Synthesizes ATP from ADP and inorganic phosphate.
- Hypothesis: Mechanism explained by the chemiosmotic hypothesis.
ATP Production
- NADH Oxidation: Produces 3 molecules of ATP.
- FADH2 Oxidation: Produces 2 molecules of ATP.
Importance of Oxygen in Respiration
- Limited Role: Oxygen’s role is primarily in the terminal stage of respiration.
- Vital Presence: Vital for driving the entire process by acting as the final hydrogen acceptor.
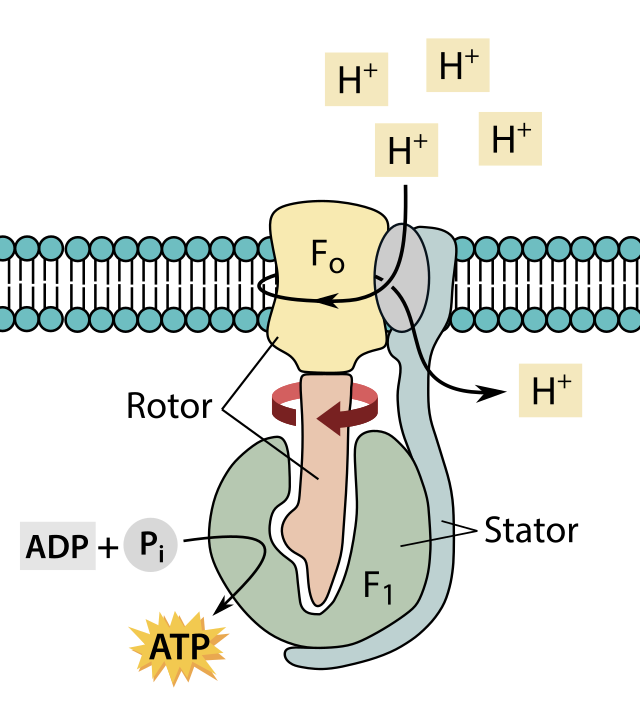
THE RESPIRATORY BALANCE SHEET
Overview
- Objective: Calculate the theoretical net gain of ATP for every glucose molecule oxidized.
- Assumptions:
- Sequential, orderly pathway: Glycolysis, TCA cycle, and ETS pathway in succession.
- Transfer of NADH to mitochondria for oxidative phosphorylation.
- No utilization of intermediates for synthesizing other compounds.
- Sole respiration of glucose; no alternative substrates entering the pathway.
- Limitations: Real-life pathways are simultaneous, substrate entry/withdrawal dynamic, and enzymatic rates are controlled by multiple factors.
Theoretical Net Gain
- Aerobic Respiration:
- Assumptions-Based Net Gain: 38 ATP molecules for one glucose molecule.
- Considerations: Sequential functioning, controlled substrate flow, and no diversion of intermediates.
Comparison with Fermentation
- Fermentation vs. Aerobic Respiration:
- Partial vs. Complete Breakdown:
- Fermentation: Partial breakdown of glucose.
- Aerobic Respiration: Complete degradation to CO2 and H2O.
- ATP Production:
- Fermentation: Net gain of only two ATP molecules per glucose degraded to pyruvic acid.
- Aerobic Respiration: Generates many more ATP molecules under aerobic conditions.
- NADH Oxidation:
- Fermentation: Slow oxidation of NADH to NAD+.
- Aerobic Respiration: Vigorous oxidation of NADH to NAD+.
- Partial vs. Complete Breakdown:
Appreciating the Living System
- Dynamic Pathways:
- Pathways work simultaneously, not sequentially.
- Substrates enter/exit pathways dynamically.
- Utilization of ATP:
- ATP is used as needed, and enzymatic rates are controlled by multiple factors.
- Exercise Purpose:
- Appreciate the efficiency of the living system in energy extraction and storage.
AMPHIBOLIC PATHWAY
Overview
- Glucose as the Primary Substrate:
- Glucose is the preferred substrate for respiration.
- All carbohydrates are usually converted into glucose before entering the respiratory pathway.
Entry Points for Different Substrates
- Points of Entry:
- Glucose: Primary substrate entering the respiratory pathway.
- Fats: Break down into glycerol and fatty acids, with entry after degradation to acetyl CoA.
- Proteins: Degraded by proteases, and amino acids enter the pathway at various stages (as pyruvate, acetyl CoA, or within Krebs’ cycle).
Catabolism vs. Anabolism
- Traditional Understanding:
- Respiration seen as a catabolic process, and the respiratory pathway as catabolic.
- Catabolism involves the breakdown of substrates.
Amphibolic Nature of the Respiratory Pathway
- Dual Role in Breakdown and Synthesis:
- Fatty Acids:
- Breakdown to acetyl CoA during respiration.
- Acetyl CoA is withdrawn for fatty acid synthesis.
- Proteins:
- Involved in both breakdown and synthesis.
- Recognition of Amphibolic Nature:
- The respiratory pathway plays a role in both catabolism and anabolism.
- Recognized as an amphibolic pathway.
- Fatty Acids:
RESPIRATORY QUOTIENT (RQ)
Overview
- Definition:
- The respiratory quotient (RQ) or respiratory ratio is the ratio of the volume of CO2 evolved to the volume of O2 consumed during aerobic respiration.
Calculation of RQ
- Formula:
- RQ=Volume of CO2 evolved Volume of O2 consumed RQ =Volume of O2 consumed volume of CO2 evolved
Dependence on Respiratory Substrate
- Carbohydrates:
- RQ is 1 when carbohydrates are completely oxidized.
- Example: C6H12O6+6O2→6CO2+6H2O+Energy
- RQ=6CO2/6O2=1
- Fats:
- RQ is less than 1 when fats are used.
- Example (Tripalmitin): C51H98O6+145O2→102CO2+98H2O+Energy
- RQ=102CO2/145O2≈0.7
- Proteins:
- RQ for proteins is about 0.9.
Importance
- Multiple Substrates:
- Living organisms often use a combination of respiratory substrates.
- Pure proteins or fats are rarely used as sole respiratory substrates.